In Part 1, we differentiate between (1) tidal-related currents in shallow marine waters and (2) purely gravitational sedimentary currents. We could add that the former are periodic, as they are controlled by the gravitational fields of the Moon and the Sun on ocean water as the Earth rotates, whereas the latter are ‘spontaneous’ currents driven by the gravitational field of the Earth on sediments and rocks, with unpredictable duration and spatial impact.
Both types of currents erode and deposit sediments on the seabed, but these are not the only types of currents in the ocean that can do so. Thermohaline currents can also achieve this. These currents are movements of ‘huge masses’ of seawater driven by gravity. As their name indicates, temperature (thermo) and salinity (haline) of seawater are the key elements promoting water movement. Simply put: the colder and saltier the water, the denser it becomes, and eventually the gravitational field of the Earth will move it to a position closer to the center of the Earth; that is, to the seabed (see key aspects of thermohaline currents in Table 1).
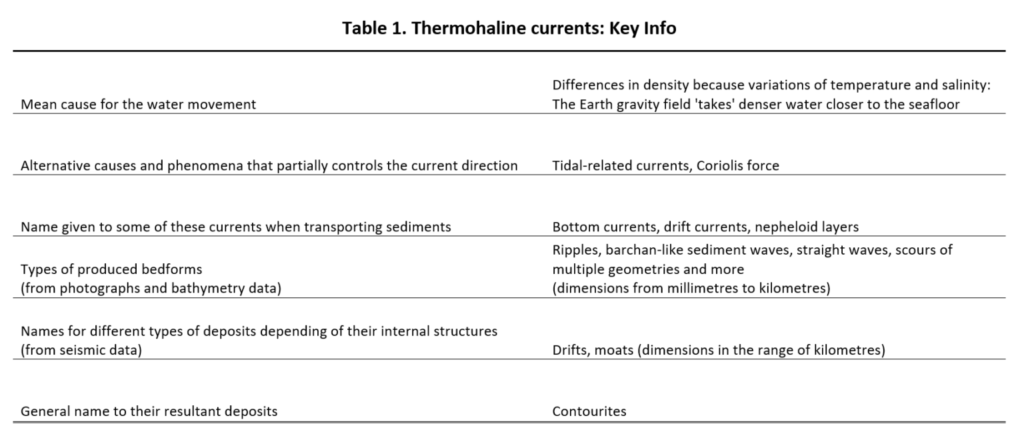
Thermohaline currents occur at any water depth. They can begin at the sea surface, as in the so-called “dense shelf water cascading process” (Canals et al., 2006), and have been observed in the deepest parts of the ocean, such as in subduction zone trenches (Thomson et al., 2010). Thermohaline currents are still not well understood, as they are connected to a complex system that integrates the dynamics of the atmosphere and the ocean. The combined action of wind stress and the Coriolis effect on the sea surface produces a series of large vortices known as oceanic gyres. These gyres are also connected to the formation of global upwelling and sinking currents that reach the seabed (see Figure 1).
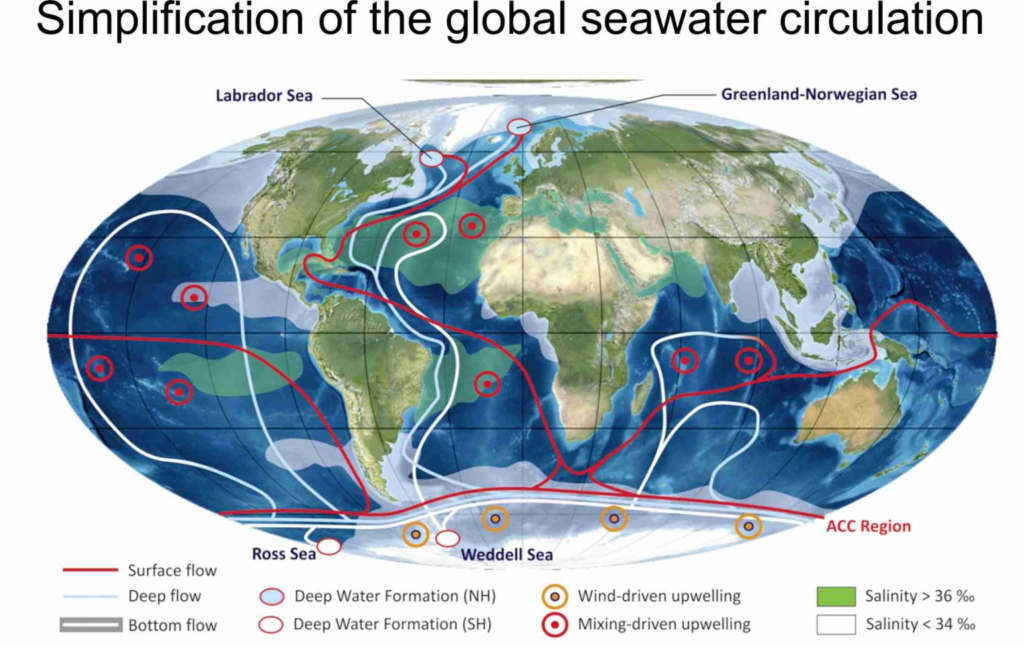
Figure 1 Global thermohaline circulation This Mollweide projection of Earth shows a simplification of global surface and subsurface currents and the areas where water sinks (deep water formation) or rises (upwelling) to the surface due to density differences. Source: Rebesco et al. 2014.
If we were to dive to abyssal depths where thermohaline currents occur, we would see ripples and grooves of any kind (see Table 1). However, on a much larger scale, these currents create stratified geometries that may be similar to some submarine channel systems formed by episodic gravity-driven currents caused by sediment and rock collapses in relatively close coastal areas. Thermohaline currents also create channels and deposits of bank-like features analogous to channel dikes connected to continental margins. These are comparatively much larger and typically formed by finer sediments.
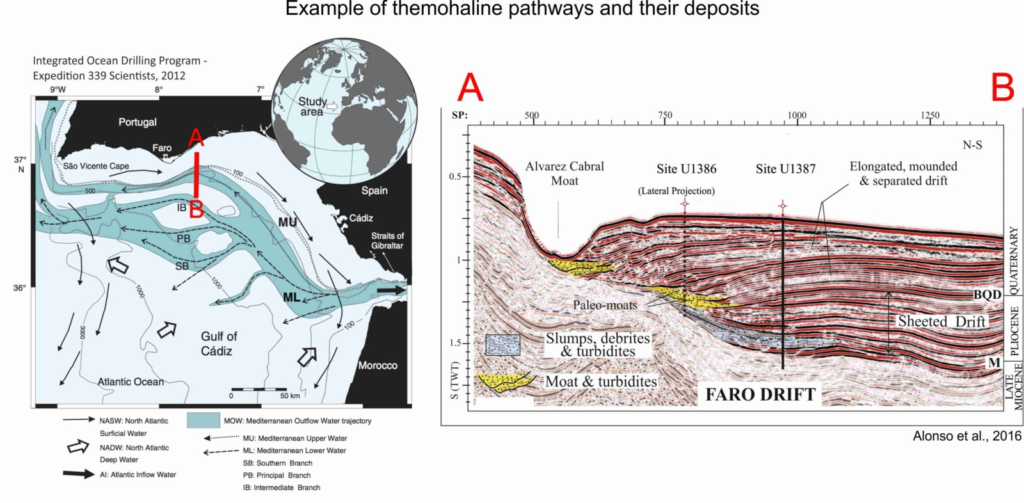
Figure 2 The pathways of thermohaline currents in the Gulf of Cádiz. The figure on the left shows the continents in black and the ocean in blue. In dark blue, the thermohaline channels where Mediterranean currents move westward. The figure on the right shows a seismic section of the northernmost channel and the dike-like deposit created by the thermohaline current, known as Faro Drift (A-B in the previous figure).
Some thermohaline channels are connected both to continental margin channels and controlled by regional tidal pathways. One of the most studied areas of this type is the Gulf of Cádiz (Figure 2).
One can imagine how important these thermohaline currents can be in the evolution of global climate. It is thought that these currents play a crucial role in controlling global climate. However, their impact on oceanic physical and chemical processes is not well understood. Therefore, the effects of anthropogenic climate change on thermohaline currents and how they interact with geological and biological systems in the deep sea are still under discussion. Research is key.
More on the links between thermohaline currents and global climate:
https://easac.eu/publications/details/a-sea-of-change-europes-future-in-the-atlantic-realm
References
Alonso, B., Ercilla, G., Casas, D., Stow, D.A., Rodríguez-Tovar, F.J., Dorador, J., and Hernández-Molina, F.J., 2016. Contour vs. gravity flow deposits of the Faro Drift from the Pleistocene (Gulf of Cádiz): Sedimentological and mineralogical approaches. Marine Geology, 377, pp.77-94.
Canals, M., Puig, P., de Madron, X.D., Heussner, S., Palanques, A., and Fabres, J., 2006. Submarine canyon outflow. Nature, 444(7117), pp.354-357.
Integrated Ocean Drilling Program - Expedition 339 Scientists, 2012. Mediterranean outflow: environmental significance of Mediterranean outflow water and its global implications. IODP Prel. Rept., 339. doi:10.2204/ iodp.pr.339.2012.
Rebesco, M., Hernández-Molina, F.J., Van Rooij, D., and Wåhlin, A., 2014. Contourites and associated sediments controlled by deep-water circulation processes: state of the art and future considerations. Marine Geology, 352, pp.111-154.
Thomson, R.E., Davis, E.E., Heesemann, M., and Villinger, H., 2010. Long-term episodic bottom currents observed in the Central America Trench: Evidence for tide-induced turbidity flows. Journal of Geophysical Research: Oceans, 115(C10).