Most of us are aware of the existence of waves and tides. We can see them along our coastlines, and although we may not pay much attention to them, we also know that waves and tides move the sediment resting on the seabed: sand, mud, shells, and… plastics.
We can all observe ripples and small channels on beaches or estuaries created by the movement of water from waves and tides. If we dive a few meters below sea level, we can see some morphologies that are the result of tidal currents and waves, such as dunes and submarine ridges (Figure 1).
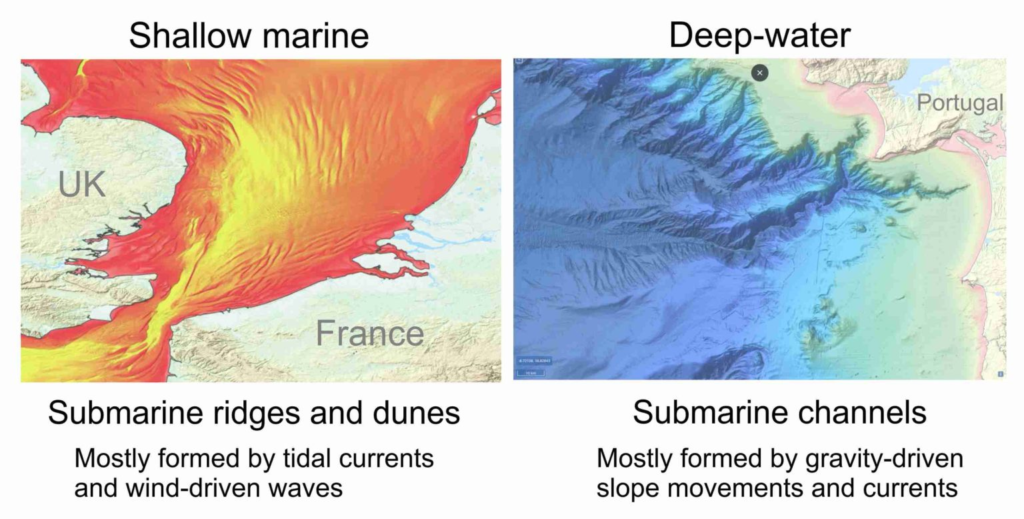
Figure 1 Seafloor morphology: shallow marine vs. deep waters. The shallow seafloor is controlled by tidal currents and wind-driven currents. These typically produce submarine bars and dunes (see the image on the left). The deep seafloor is controlled by gravity-driven currents. Submarine channels are likely the most notable morphologies resulting from these types of currents (see the image on the right). Images modified from www.EMODnet.eu.
But is that all there is to the seafloor? What happens if we move to deeper parts of the seafloor? Many of us have seen maps representing the general morphology of Earth’s seafloor (https://earthobservatory.nasa.gov/images/87189/seafloor-features-are-revealed-by-the-gravity-field). These maps show a very rugged seafloor surface. Using the same terminology created for our continents, we see valleys, mountains, volcanoes, and enormous depressions. And things get really interesting when we look at high-resolution images from cutting-edge bathymetric surveys of these deep ocean floors. We see morphologies that resemble… rivers! Yes, they look like rivers, but they are called ‘submarine channels’ (Figure 1). But how is this possible if we are already underwater… rivers within the ocean? Not exactly… The structures resembling river shapes on the seafloor are formed by sediments moving downhill.
We know of several scenarios where relatively large volumes of sediment regularly fall through the water column and move downhill on the seafloor solely due to the effect of the Earth’s gravitational field. The most common are: (1) the input of sediment from river outflows into the ocean, (2) the collapse of accumulated sediments at the heads of channels or along their first few kilometers, (3) the input of accumulated sediments at the heads of channels by wave and tide-related currents, and (4) the collapse of relatively large portions of the seafloor due to earthquakes. These scenarios result in a wide range of mixtures of different types of rocks and particle sizes (including human-made debris!).
Whatever the mixture of solid materials, the sediment particles that fall also displace the surrounding water, pushing it outward and dragging it in the same direction as the flow. This is crucial because there comes a point when the sediment and parts of the surrounding water begin to move in the same direction; a kind of mixing of water flow and sediment moving independently in the water. You can call this type of current: ‘gravity-driven sediment flow’; but many prefer to simply call it ‘turbidity flow or turbidity current.’ You will see that flow and current are terms used interchangeably, but for studies of ‘flow dynamics’, “flow” is the preferred term.
In the end, both underwater currents and turbidity currents, as well as flows in rivers, affect the substrate in similar ways: they erode and deposit sediment. Water, due to its viscosity, can push and pull particles like mineral fragments or shells as it moves. The latter can also collide with other particles, pushing and displacing them to other locations.
There are also a number of ‘flow phenomena’ that occur in both rivers and submarine channels with similar consequences. Hydraulic jumps are one of the most effective in eroding and depositing sediment among these phenomena. The hydraulic jump results from the complex interaction between the gravitational field and the physical properties of water and solid particles. Simplifying, if a fast-moving flow going downhill is forced to decelerate ‘too quickly’, it can develop a localized burst or a so-called jump. It’s like an upside-down waterfall. The jump resembles a typical breaking wave on a beach, but don’t be mistaken, the fluid dynamics are entirely different (Figure 2-1). The water moves upward in these ‘jumps’, creating a negative pressure in the area of the floor beneath the burst. It behaves like a vacuum cleaner, trying to suck up what’s on the floor. These hydraulic jumps can create large holes (grooves) in the seafloor and incorporate tons of new sediment into an ongoing flow (Figure 2-2). Hydraulic jumps are extremely difficult to observe in underwater environments, but recent research efforts in submarine channels connected to deltas have recorded sediment movement that matches the expected movement of sediments along downstream sections (Figure 2-3).
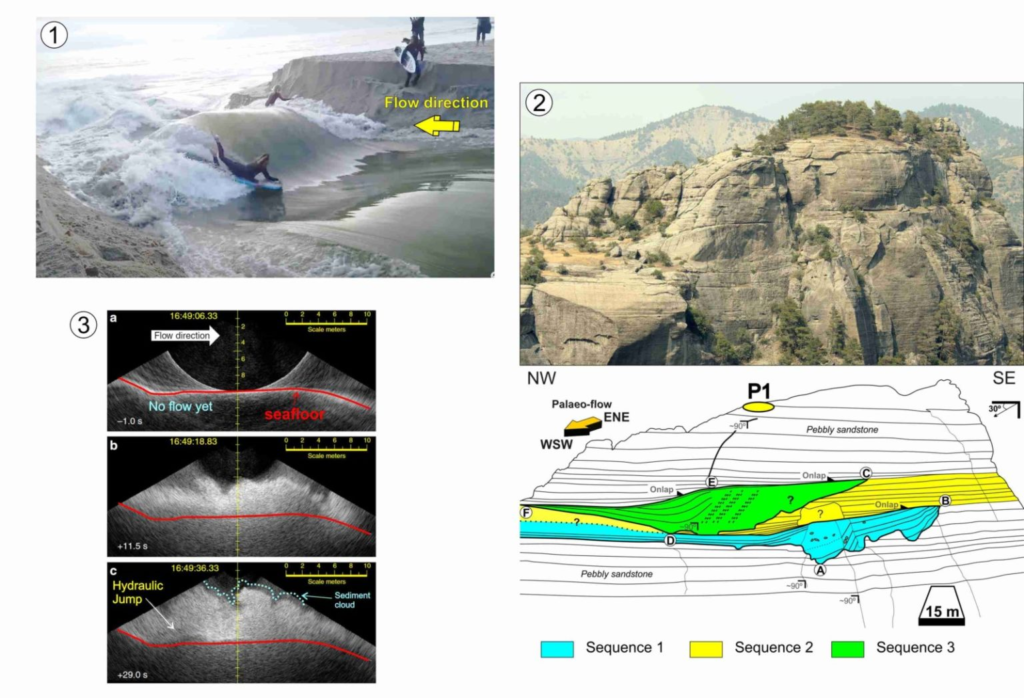
Figure 2 Hydraulic jumps in flow and their effects on the sedimentary record. 1) A surfer rides atop a hydraulic jump formed by a strong current coming from a lagoon to the right of the image (see the full video here: https://youtu.be/eDmoXkF-g9I?t=278). 2) The fill of the Alikayasi canyon, part of a submarine channel that existed 12 million years ago, shows groove and fill architectures that can be explained by interpreting the occurrence of powerful hydraulic jumps (see ongoing research here: https://eartharxiv.org/repository/view/1298/). 3) These images show three snapshots of a flow that occurred in submarine channels connected to the Squamish River delta. An acoustic Doppler current profiler (ADCP) recorded a sediment burst that formed a cloud with 2D morphologies similar to hydraulic jumps in aerial environments (see Hughes Clarke, 2016).
In summary, there are geomorphologies on the deep seafloor similar to continental rivers and produced by processes that share some similarities, but the differences can also be significant. We still define many of the processes that transport and deposit sediments in the deep ocean based on a good amount of assumptions. We need to continue research efforts focused on studying the deeper, darker parts of our oceans. In the next article, we will discuss another type of gravity-driven current in the ocean that shapes the deepest parts of the seafloor: thermohaline currents.
References: Hughes Clarke, J.E.H., 2016. First wide-angle view of channelized turbidity currents links migratory cyclic steps to flow characteristics. Nature communications, 7(1), pp.1-13.